Experimental Study of the Raman Effect:
The Raman effect has been extensively investigated by a large number of workers. The general principle used in these researches is to illumine the substance under test with an intense monochromatic source of light and photograph the scattered radiation by means of a spectrograph arranged in a transverse direction. But the technical details vary according to the nature of the substance studied, i.e. solid, liquid, or gas, the chief aim being to obtain the best and quick results.
Apparatus: The original simple arrangement of Raman was not quite efficient and required very long exposures, about 100 hours and more, to obtain good records of the Raman spectrum. Hence improvements were made as regards the container of the substance, the source of radiation, filters, spectrograph, etc.
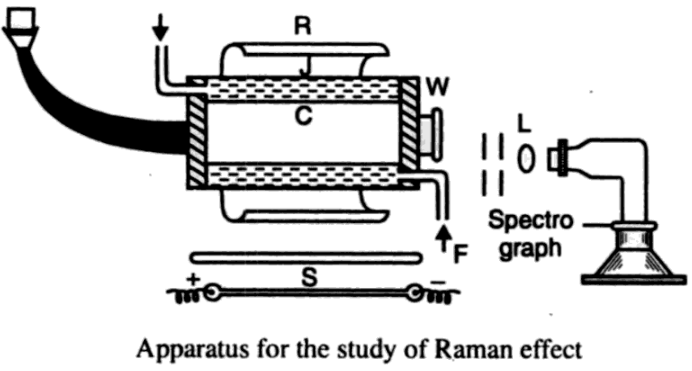
The apparatus shown above is the one, first designed by Wood and is now ordinarily used in the study of the Raman effect in liquids.
The container C of the liquid to be investigated, called the Raman tube, consists of a glass tube of about 1 or 2 cm in diameter, and 10 to 15 cm long, one end of which is drawn out into the shape of a horn and blackened outside to provide a suitable background, the other end being closed with an optically plane glass plate constituting the window W through which the scattered light emerges. The container is surrounded by a water jacket in which cold water is circulated to percent overheating of the liquid in the Raman tube due to the proximity of the exciting arc.
An ideal source S would be light from a helium discharge tube filtered by nickel oxide glass, giving a strictly monochromatic line of wavelength 3888 Å. But on account of the many technical difficulties involved in the construction and manipulation of this source, it is not widely used. The source ordinarily employed is the mercury arc, the next best available, from which it is possible to get single wavelengths by the use of suitable filters. Thus, for instance, to obtain a 4358 Å line, a slightly acidulated quinine sulfate solution contained in a novial glass vessel is used as a filter, which cuts off all the other lines except the 4358 Å. To get the 4046 Å line, a solution of iodine in carbon tetrachloride contained in a novial glass cell is found to be a very satisfactory filter. The filter solution may be arranged either to surround the Raman tube or in front of the arc. The mercury arc is placed as close to the Raman tube as possible, which results in a large intensity of the incident light. A semi-cylindrical aluminium reflector R, enhances the intensity of illumination still further.
The chief features of a spectrograph, suited for the study of Raman spectra are:
- Large light gathering power.
- Special prisms of high resolving power.
- A short focus camera. A lens L in front of the window W directs the scattered radiation upon the slit of the spectrograph, which is carefully aligned along the axis of the Raman tube and screened from the direct rays of the arc. The more intense Raman lines of a liquid such as CCl2 can be photographed in about an hour with a small spectrograph, but the recording of the complete spectrum may require up to ten to fifteen hours, depending largely on the intensity of the incident light, the speed of the spectrograph and the intrinsic brilliance of the Raman lines. It may be noted that instruments of high resolving power such as gratings are not used with advantage, on account of their poor luminosity which necessitates long exposures.
Certain modifications in the apparatus are necessary for the excitation of the Raman effect in solids and gases. In the case of solids which are available as large and transparent blocks, like gypsum, quartz, etc., a container is not required and the light from the arc can be directly focussed on the substance with a large condensing lens. With solids that are in the form of loose crystal powders, the Raman effect can be obtained as was first shown by Bear and Menzies, by reflecting light from the crystal surfaces. But special precaution has to be taken to avoid the masking effect due to a large amount of direct light coming out of the container by repeated reflection at the crystal faces and entering the spectrograph. To achieve this, two techniques have been used: one the use of complementary filters as developed by Ananthakrishnan at the Raman laboratory, and the other a special type of spectrograph with two parts each part having a prism and two lenses with a common slit in between the two, devised by Billroth, Kohlrausch, and Reitz in Germany. Both give very good results with crystal powders and the latter even with very small quantities of the substance.
In the case of gases, the intensity of the light scattered is normally very weak, but the difficulty has been overcome by intense illumination of the gas under high pressure and the use of a spectrograph of large light-gathering power. Wood employed a very long tube of HCl gas and obtained its Raman spectrum at atmospheric pressure using a specially made mercury arc which was placed in contact with the gas tube, hollow cylindrical reflectors enclosing both of them. The illumination produced in this way was very intense as the light from the arc was reflected back and forth between the walls of the reflectors. Rosetti was the first to develop the technique of exciting the Raman effect in gases under high pressure, which shortened considerably the time of exposure. He used a thick-walled quartz tube 20 cm long and 2.2 cm internal diameter, which could stand pressures of 10 to 15 atmospheres. With the 2537 Å line of the mercury arc, he was able to obtain the Raman spectra of several gases under pressure. Bhagavantam has constructed a Raman tube for gases that can stand pressure up to 50 atmospheres. It is made of transparent silica and enclosed in an outer steel tube for protection. He has been able to obtain with his arrangement good spectrographs of Raman spectra of gases in about 40 or 50 hours time of exposure using the 3650 Å, 4046 Å, and 4358 Å mercury lines.
Comments (No)